Methods
- Terahertz scanning tunneling microscopy
- Reflective echelon mirror
- Chirp-pulse up-conversion / Time-lens method
- Broadband terahertz spectroscopy
Terahertz scanning tunneling microscopy
Scanning tunneling microscopy (STM) is a powerful technique to measure nanoscale physical properties of materials whereas the time resolution of the measurement is typically limited to several milliseconds that is determined by the low-noise current amplifier used to observe tiny tunneling current induced between a tip and a sample. The use of ultrashort pulsed lasers and terahertz pulses overcomes this difficulty to increase the temporal resolution to the picosecond timescales, where dynamical motion of electrons and phonons appear. We shine two pulses on the STM tip and tune the interval to acquire the time-dependent modulation of the tunneling current induced either by optical or terahertz pulses. In our group, we are developing various types of such terahertz STMs for visualizing and manipulating the invisibles in the materials surface.
<For more information, please see the followings>
Ikufumi Katayama et al., “Investigation of ultrafast excited-state dynamics at the nanoscale with terahertz field-induced electron tunneling and photon emission,” Journal of Applied Physics 133, 110903 (2023).
<Our related papers>
Jun Takeda et al., “Nanoscale electron manipulation in metals with intense THz electric fields,” J. Phys. D: Appl. Phys. 51 103001 (2018).
Katsumasa Yoshioka et al., “Real-space coherent manipulation of electrons in a single tunnel junction by single-cycle terahertz electric fields,” Nature Photon. 10, 7620765 (2016).
Reflective echelon mirror
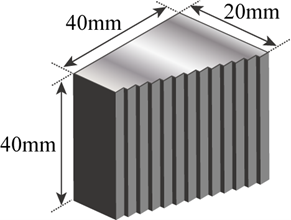
Ultrafast and terahertz spectroscopy normally uses repeated measurements of the time-domain information with changing the delay time between the pump and the sample. Because this strategy assumes that the phenomenon is repeatable, it is hard to apply this method to irreversible phenomena (for examples, laser ablation, laser induced damage, photoinduced phase change, chaotic phenomena, noise spectrsocopy, chemical reactions etc.) that could not be repeated easily with the similar experimental conditions. To reveal and understand the ultrafast dynamics even in such irreversible phenomena, we fabricated a reflective echelon mirror as shown in the figure to generate a train of many probe pulses (typically ~400) from a single laser shot. We focus the reflection of the echelon to the sample, and record the temporal information using a camera to capture the pump induced changes of the transmittance as a function of time within a single laser shot. By using this system, we could visualize various ultrafast waveforms including terahertz and pump-probe traces within a single shot.
<For more information, please see the followings>
Ikufumi Katayama and Ryo Tamaki, “Singleshot and high-sensitivity detection of terahertz waveforms using phase-offset electro-optic sampling and an echelon mirror,” Proc. SPIE 12406, Real-time Measurements, Rogue Phenomena, and Single-Shot Applications VIII, 1240603 (14 March 2023).
<For getting echelons>
Manufacturer: Sodick F.T. (in Japanese)
Please contact us, or directly consult Sodick Co., Ltd. for details.
<Our related papers>
R. Tamaki, T. Kasai, G. Asai, D. Hata, H. Kubo, Y. Takigawa, J. Takeda and I. Katayama, “Pulse-to-pulse Detection of Terahertz Radiation Emitted from the Femtosecond Laser Ablation Process”, Opt. Exp. 30, pp. 23622-23630 (2022).
G. Asai, D. Hata, S. Harada, T. Kasai, Y. Arashida, and I. Katayama, “High-throughput terahertz spectral line imaging using an echelon mirror”, Opt. Exp. 29, pp. 3515-3523 (2021).
Please also check the combination of singleshot terahertz spectroscopy with Rice Advanced Magnet with Broadband Optics (RAMBO).
Chirp-pulse up-conversion / Time-lens method
Another experimental technique that are capable of measuring the irreversible phenomena is to use the time-encoding technique where the chirped probe pulses are used to map the temporal information to the wavelength of the laser. In comparison with the echelon based technique, this method is powerful because the imaging optics is not required in the measurement system since the temporal information is written in the wavelength, which is the internal degree of freedom of the light pulses. This enables us to extend the applicability of the single-shot technique to fiber-based technologies that are more robust and stable than the free space optics. However, there was a severe drawback of the technique where the signal waveform is strongly distorted in the ultrafast timescale, which prevent the applicability of the technique to wider areas. To circumvent this difficulty and extending the applications of singleshot spectroscopy, we used so-called time-lens method to the time-encoding technique, where the chirp pulse up-conversion spectroscopy is utilized to precisely map the temporal information to the wavelength. We call such a technology “terahertz oscilloscope,” because we can visualize the terahertz waveforms without severe distorsions.
<For more information, please see the followings>
R. Tamaki, M. Suzuki, J. Takeda, and I. Katayama, “Chirped-Pulse Up-Conversion Spectroscopy Applicable to Terahertz Waveform Detection in Time-Domain,” in The International Conference on Ultrafast Phenomena (UP) 2022, Technical Digest Series (Optica Publishing Group, 2022), paper Tu4A.46.
<Our related papers>
Masataka Kobayashi et al., “Pulse-to-pulse ultrafast dynamics of highly photoexcited Ge2Sb2Te5 thin films,” Jpn. J. Appl. Phys. 62, 022001, (2023).
M. Kobayashi, Y. Arashida, G. Yamashita, E. Matsubara, M. Ashida, J. A. Johnson, and I. Katayama, “Fast-Frame Single-shot Pump-Probe Spectroscopy with Chirped-Fiber-Bragg Gratings”, Opt. Lett. 44, 163-166 (2019).
Broadband terahertz and coherent phonon spectroscopy
Terahertz region (0.1-10 THz) is one of the important frequency range to visualize the materials properties and their dynamics. Terahertz technology often uses ultrashort pulses to generate and detect the terahertz waves, and the bandwidth of the terahertz pulses are limited by the inverse of Fourier transformation and the absorption of the material used in the setup. In our group, we use the reflection geometry of the photoconducting antenna to generate and detect the broadband terahertz waves by avoiding the absorption of the terahertz pulses and to reach the bandwidth that is solely determined by the pulse duration of the excitation laser. We use a 15-fs laser pulses to generate and detect terahertz waves, and obtained the broad bandwidth from 0.1 THz to 10 THz. This bandwidth could bridge the frequency range of FTIR and microwave spectroscopy, and thus, could be very useful for various researches on material characterization such as organic materials, 2D-materials, ferroelectric materials, and meteorites.
<For more information, please see the followings>
T. Kawano, I. Katayama, J. Ohara, M. Ashida and J. Takeda, “Intermolecular THz Vibrations Relevant to Optically and Thermally Induced Magnetic Phase Transitions in the Strongly Correlated Organic Radical TTTA”, J. Phys. Soc. Jpn. 83, 014713: pp. 1-6 (2014).
<Our related papers>
I. Katayama, R. Akai, M. Bito, E. Matsubara, and M. Ashida, “Electric Field Detection of Phase-Locked Near-Infrared Pulses Using Photoconductive Antenna,” Opt. Exp. 21, pp. 16248-16254 (2013).